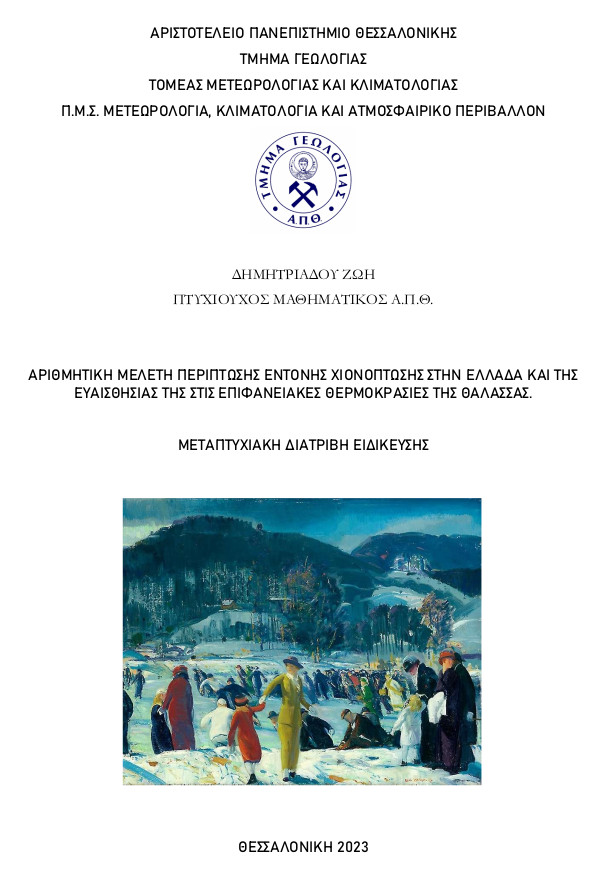
Αριθμητική μελέτη περίπτωσης έντονης χιονόπτωσης στην Ελλάδα και της ευαισθησίας της στις επιφανειακές θερμοκρασίες της θάλασσας. = Numerical Study of An Intense Snow Fall In Greece And Its Sensitivity To Sea Surface Temperatures.
Περίληψη
This thesis deals with the numerical, synoptic and dynamic analysis of the intense snowfall event that occurred in Greece on February 13-17, 2021, and was named "Medea". A major aim of this work was the study of its sensitivity to the sea surface temperatures. This event was one of the most intense snowfall phenomena of the last 20 years in Greece. It caused the loss of three lives, the destruction of property, the interruption of electricity, heating, telephony, and the Internet, as well as the obstruction of all kinds of transport (highways, sea transport and flight cancellations). The phenomenon affected many parts of the country, but mainly the capital, which did not have the infrastructure to manage such an intense snowfall. This work is pioneer in the study of the phenomenon of snowfall with the influence of the sea in the Greek region and especially the influence of the Aegean Sea and its surface temperatures on the intense phenomena and snowfall in Greece. It also attempts to fill the gap that exists in the international literature in the study of the influence of sea surface temperatures on snowfall in the Eastern Mediterranean. The synoptic and dynamic analysis of the snowfall event was initially performed. Secondly, experiments were carried out for the optimal simulation of the phenomenon using the nonhydrostatic Weather and Research Forecasting (WRF) numerical model. Also, a series of experiments were carried out to study the influence of sea surface temperatures on the intense snowfall event. The data used for the analysis of the phenomenon included the available surface and upper air observations from the meteorological stations of the Hellenic National Meteorological Service (HNMS), data collection from the media, operational gridded analyses of the European Centre for Medium-Range Weather Forecasts (ECMWF) and weather charts from the United Kingdom Meteorological Office (UKMet). Furthermore, the sea surface temperatures were based on the high-resolution daily gridded analyses of the National Centers for Environmental Prediction (NCEP) and lower resolution gridded climatology. The synoptic/dynamic analysis showed that this phenomenon can be considered as a case of lake/sea effect snow. The optimum WRF simulation of the event was produced by the combination of the Yonsei University boundary layer parameterization with explicitly resolved cumulus convection. In the last part of this thesis, it was shown that local sea surface temperature anomalies up to ±3°C in the Aegean Sea are not able to greatly affect a phenomenon like "Medea". Nevertheless, the decrease in sea temperatures (which also corresponds to the climatic data of sea surface temperatures) tends to reduce the intensity of the phenomenon with smaller precipitation and snowfall amounts. On the contrary, the increase in sea surface temperatures amplifies the phenomenon to a small extent.
Πλήρες Κείμενο:
PDFΑναφορές
Arakawa, A., Jung, J.-H., & Wu, C.-M. (2011). Toward unification of the multiscale modeling of the atmosphere. Atmospheric Chemistry and Physics, 11(8), 3731–3742. https://doi.org/10.5194/acp-11-3731-2011
Arakawa, A., & Lamb, V. R. (1977). Computational Design of the Basic Dynamical Processes of the UCLA General Circulation Model. In J. Chang (Ed.), Methods in Computational Physics: Advances in Research and Applications (Vol. 17, pp. 173–265). Elsevier. https://doi.org/10.1016/B978-0-12-460817-7.50009-4
Baldwin, M. E., Kain, J. S., & Kay, M. P. (2002). Properties of the Convection Scheme in NCEP’s Eta Model that Affect Forecast Sounding Interpretation. Weather and Forecasting, 17(5), 1063–1079. https://doi.org/10.1175/1520-0434
Betts, A. K. (1986). A new convective adjustment scheme. Part I: Observational and theoretical basis. Quarterly Journal of the Royal Meteorological Society, 112(473), 677–691. https://doi.org/10.1002/qj.49711247307
Betts, A. K., & Miller, M. J. (1986). A new convective adjustment scheme. Part II: Single column tests using GATE wave, BOMEX, ATEX and arctic air-mass data sets. Quarterly Journal of the Royal Meteorological Society, 112(473), 693–709. https://doi.org/10.1002/qj.49711247308
Demirtaş, M. (2022). A lake‐effect snowstorm over southern Europe with upstream blocking in early January 2017. Weather, wea.4192. https://doi.org/10.1002/wea.4192
Doswell, C. A., Davies-Jones, R., & Keller, D. L. (1990). On Summary Measures of Skill in Rare Event Forecasting Based on Contingency Tables. Weather and Forecasting, 5(4), 576–585. https://doi.org/10.1175/1520-0434
Fernández‐González, S., Valero, F., Sánchez, J. L., Gascón, E., López, L., García‐Ortega, E., & Merino, A. (2015). Numerical simulations of snowfall events: Sensitivity analysis of physical parameterizations. Journal of Geophysical Research: Atmospheres, 120(19). https://doi.org/10.1002/2015JD023793
Freitas, S. R., Grell, G. A., & Li, H. (2021). The Grell–Freitas (GF) convection parameterization: Recent developments, extensions, and applications. Geoscientific Model Development, 14(9), 5393–5411. https://doi.org/10.5194/gmd-14-5393-2021
Fritsch, J. M., & Chappell, C. F. (1980). Numerical Prediction of Convectively Driven Mesoscale Pressure Systems. Part I: Convective Parameterization. Journal of the Atmospheric Sciences, 37(8), 1722–1733. https://doi.org/10.1175/1520-0469
Gandin, L. S., & Murphy, A. H. (1992). Equitable Skill Scores for Categorical Forecasts. Monthly Weather Review, 120(2), 361–370. https://doi.org/10.1175/1520-0493
Ghafarian, P., Delju, A. H., Tajbakhsh, S., & Penchah, M. M. (2021). Simulation of the role of Caspian Sea surface temperature and air temperature on precipitation intensity in lake-effect snow. Journal of Atmospheric and Solar-Terrestrial Physics, 225, 105777. https://doi.org/10.1016/j.jastp.2021.105777
Ghafarian, P., Pegahfar, N., & Owlad, E. (2018). Multiscale analysis of lake-effect snow over the southwest coast of the Caspian Sea (31 January–5 February 2014). Weather, 73(1), 9–14. https://doi.org/10.1002/wea.3055
Glossary of Meteorology. (2020, November 2). https://glossary.ametsoc.org/wiki/Thermal_low
Grell, G. A., & Dévényi, D. (2002). A generalized approach to parameterizing convection combining ensemble and data assimilation techniques: PARAMETERIZING CONVECTION COMBINING ENSEMBLE AND DATA ASSIMILATION TECHNIQUES. Geophysical Research Letters, 29(14), 38-1-38–4. https://doi.org/10.1029/2002GL015311
Grell, G. A., & Freitas, S. R. (2014a). A scale and aerosol aware stochastic convective parameterization for weather and air quality modeling. Atmospheric Chemistry and Physics, 14(10), 5233–5250. https://doi.org/10.5194/acp-14-5233-2014
Grell, G., & Freitas, S. (2014b). Further development and initial evaluations of the aerosol dependence modeled by the Grell and Freitas convective parameterization. 8807. https://ui.adsabs.harvard.edu/abs/2014EGUGA..16.8807G
Hong, S.-Y., Noh, Y., & Dudhia, J. (2006). A New Vertical Diffusion Package with an Explicit Treatment of Entrainment Processes. Monthly Weather Review, 134(9), 2318–2341. https://doi.org/10.1175/MWR3199.1
Hong, S.-Y., & Pan, H.-L. (1996). Nonlocal Boundary Layer Vertical Diffusion in a Medium-Range Forecast Model. Monthly Weather Review, 124(10), 2322–2339. https://doi.org/10.1175/1520-0493
Hoskins, B. J., McIntyre, M. E., & Robertson, A. W. (1985). On the use and significance of isentropic potential vorticity maps. Quarterly Journal of the Royal Meteorological Society, 111(470), 877–946. https://doi.org/10.1002/qj.49711147002
Janjić, Z. (1990). The Step-Mountain Coordinate: Physical Package. Monthly Weather Review, 118(7), 1429–1443. https://doi.org/10.1175/1520-0493
Janjić, Z. (1994). The Step-Mountain Eta Coordinate Model: Further Developments of the Convection, Viscous Sublayer, and Turbulence Closure Schemes. Monthly Weather Review, 122(5), 927–945. https://doi.org/10.1175/1520-0493
Janjić, Z. (1996). The surface layer in the NCEP Eta Model. Proceedings of the 11th conference on numerical weather prediction, 19–23 August 1996, Norfolk, VA, 354–355
Janjić, Z. (2000). Comments on “Development and Evaluation of a Convection Scheme for Use in Climate Models.” Journal of the Atmospheric Sciences, 57(21), 3686–3686. https://doi.org/10.1175/1520-0469
Janjić, Z. (2002). Nonsingular Implementation of the Mellor–Yamada Level 2.5 Scheme in the NCEP Meso Model. NCEP Office Note, 436.
Kain, J. S. (2004). The Kain–Fritsch Convective Parameterization: An Update. Journal of Applied Meteorology, 43(1), 170–181. https://doi.org/10.1175/1520-0450
Kain, J. S., & Fritsch, J. M. (1990). A One-Dimensional Entraining/Detraining Plume Model and Its Application in Convective Parameterization. Journal of the Atmospheric Sciences, 47(23), 2784–2802. https://doi.org/10.1175/1520-0469
Kain, J. S., & Fritsch, J. M. (1993). Convective Parameterization for Mesoscale Models: The Kain-Fritsch Scheme. In K. A. Emanuel & D. J. Raymond (Eds.), The Representation of Cumulus Convection in Numerical Models (pp. 165–170). American Meteorological Society. https://doi.org/10.1007/978-1-935704-13-3_16
Katsafados, P., Mavromatidis, E., Papadopoulos, A., & Pytharoulis, I. (2011). Numerical simulation of a deep Mediterranean storm and its sensitivity on sea surface temperature. Natural Hazards and Earth System Sciences, 11(5), 1233–1246. https://doi.org/10.5194/nhess-11-1233-2011
Kindap, T. (2010). A severe sea-effect snow episode over the city of Istanbul. Natural Hazards, 54(3), 707–723. https://doi.org/10.1007/s11069-009-9496-7
Kottek, M., Grieser, J., Beck, C., Rudolf, B., & Rubel, F. (2006). World Map of the Köppen-Geiger climate classification updated. Meteorologische Zeitschrift, 15(3), 259–263. https://doi.org/10.1127/0941-2948/2006/0130
Laird, N. F., Walsh, J. E., & Kristovich, D. A. R. (2003). Model Simulations Examining the Relationship of Lake-Effect Morphology to Lake Shape, Wind Direction, and Wind Speed. Monthly Weather Review, 131(9), 2102–2111. https://doi.org/10.1175/1520-0493
Lolis, C. J., Bartzokas, A., & Katsoulis, B. D. (2004). Relation between sensible and latent heat fluxes in the Mediterranean and precipitation in the Greek area during winter. International Journal of Climatology, 24(14), 1803–1816. https://doi.org/10.1002/joc.1112
Matzarakis, A., Karatarakis, N., & Sarantopoulos, A. (2005). Tourism climatology and tourism potential for. Annalen Der Meteorologie, 616–619.
Mazarakis, N. (2010). Παρατηρησιακή και αριθμητική μελέτη των δυναμικών και φυσικών διεργασιών που συνδέονται με τη θερινή καταιγιδοφόρο δραστηριότητα στον ελλαδικό χώρο [Πανεπιστήμιο Πατρών, Σχολή Θετικών Επιστημών, Τμήμα Φυσικής, Τομέας Εφαρμοσμένης Φυσικής, Εργαστήριο Φυσικής της Ατμόσφαιρας]. https://doi.org/10.12681/eadd/27984
Mesinger, F., Veljovic, K., Chou, S. C., & Lyra, J. G., and A. (2016). The Eta Model: Design, Use, and Added Value. In Topics in Climate Modeling. IntechOpen. https://doi.org/10.5772/64956
Miglietta, M. M., Moscatello, A., Conte, D., Mannarini, G., Lacorata, G., & Rotunno, R. (2011). Numerical analysis of a Mediterranean ‘hurricane’ over south-eastern Italy: Sensitivity experiments to sea surface temperature. Atmospheric Research, 101(1), 412–426. https://doi.org/10.1016/j.atmosres.2011.04.006
Niziol, T. A. (1987). Operational Forecasting of Lake Effect Snowfall in Western and Central New York. Weather and Forecasting, 2(4), 310–321. https://doi.org/10.1175/1520-0434(1987)002<0310:OFOLES>2.0.CO;2
Noh, Y., Cheon, W. G., Hong, S. Y., & Raasch, S. (2003). Improvement of the K-profile Model for the Planetary Boundary Layer based on Large Eddy Simulation Data. Boundary-Layer Meteorology, 107(2), 401–427. https://doi.org/10.1023/A:1022146015946
Norris, J., Vaughan, G., & Schultz, D. M. (2013). Snowbands over the English Channel and Irish Sea during cold-air outbreaks: English Channel and Irish Sea Snowbands. Quarterly Journal of the Royal Meteorological Society, 139(676), 1747–1761. https://doi.org/10.1002/qj.2079
Nurmi, P. (2003). Recommendations on the verification of local weather forecasts. ECMWF technical memorandum, No. 430. https://doi.org/10.21957/Y1Z1THG5L
γPapadopoulos, A., & Katsafados, P. (2009). Verification of operational weather forecasts from the POSEIDON system across the Eastern Mediterranean. Natural Hazards and Earth System Sciences, 9(4), 1299–1306. https://doi.org/10.5194/nhess-9-1299-2009
Prezerakos, N. G., & Angouridakis, V. E. (1984). Synoptic consideration of snowfall in Athens. Journal of Climatology, 4(3), 269–285. https://doi.org/10.1002/joc.3370040305
Pytharoulis, I. (2018). Analysis of a Mediterranean tropical-like cyclone and its sensitivity to the sea surface temperatures. Atmospheric Research, 208, 167–179. https://doi.org/10.1016/j.atmosres.2017.08.009
Pytharoulis, I., Kotsopoulos, S., Tegoulias, I., Kartsios, S., Bampzelis, D., & Karacostas, T. (2016). Numerical modeling of an intense precipitation event and its associated lightning activity over northern Greece. Atmospheric Research, 169, 523–538. https://doi.org/10.1016/j.atmosres.2015.06.019
Pytharoulis, I., Tegoulias, I., Kotsopoulos, S., Bampzelis, D., Karacostas, T., & Katragkou, E. (2014). Evaluation of the operational numerical weather predictions of the WaveForUs project. Proceedings of the 12th international conference on Meteorology, Climatology and Atmospheric Physics (COMECAP2014), 28-31 May, Heraklion, Crete, Greece, Vol. 3, 96-101.
Reynolds, R. W., Rayner, N. A., Smith, T. M., Stokes, D. C., & Wang, W. (2002). An Improved In Situ and Satellite SST Analysis for Climate. Journal of Climate, 15(13), 1609–1625. https://doi.org/10.1175/1520-0442(2002)015<1609:AIISAS>2.0.CO;2
Sakallı, A. (2017). Sea surface temperature change in the mediterranean sea under climate change: A linear model for simulation of the sea surface temperature up to 2100. https://doi.org/10.15666/aeer/1501_707716
Savijärvi, H. (2015). Cold air outbreaks along a non-frozen sea channel: Effects of wind on snow bands. Meteorology and Atmospheric Physics, 127(4), 383–391. https://doi.org/10.1007/s00703-015-0370-8
Schmidlin, T. (1989). Climatic Summary of Snowfall and Snow Depth in the Ohio Snowbelt at Chardon 1. The Ohio Journal of Science, 89(4), 101–108.
Senatore, A., Mendicino, G., Knoche, H. R., & Kunstmann, H. (2014). Sensitivity of Modeled Precipitation to Sea Surface Temperature in Regions with Complex Topography and Coastlines: A Case Study for the Mediterranean. Journal of Hydrometeorology, 15(6), 2370–2396. https://doi.org/10.1175/JHM-D-13-089.1
Shi, Q., & Xue, P. (2019). Impact of Lake Surface Temperature Variations on Lake Effect Snow Over the Great Lakes Region. Journal of Geophysical Research: Atmospheres, 124(23), 12553–12567. https://doi.org/10.1029/2019JD031261
Skamarock, W. C., Klemp, J. B., Dudhia, J., Gill, D. O., Liu, Z., Berner, J., Wang, W., Powers, J. G., Duda, M. G., Barker, D. M., & Huang, X.-Y. (2019). A Description of the Advanced Research WRF Model Version 4. UCAR/NCAR. https://doi.org/10.5065/1DFH-6P97
Skamarock, W., Klemp, J., Dudhia, J., Gill, D., Barker, D., Wang, W., Huang, X.-Y., & Duda, M. (2008). A Description of the Advanced Research WRF Version 3 (p. 1002 KB) [Application/pdf]. UCAR/NCAR. https://doi.org/10.5065/D68S4MVH
Sousounis, P. J. (2003). LAKE-EFFECT STORMS. In Encyclopedia of Atmospheric Sciences (pp. 1104–1115). Elsevier. https://doi.org/10.1016/B0-12-227090-8/00195-0
Tegoulias, I. (2020). Implementation of an innovative methodology for the evaluation and optimization of the WRF model for the study of convective activity in Thessaly [Αριστοτέλειο Πανεπιστήμιο Θεσσαλονίκης (ΑΠΘ), Σχολή Θετικών Επιστημών, Τμήμα Γεωλογίας, Τομέας Μετεωρολογίας και Κλιματολογίας]. https://doi.org/10.12681/eadd/47350
Theeuwes, N. E., Steeneveld, G. J., Krikken, F., & Holtslag, A. a. M. (2010). Mesoscale modeling of lake effect snow over Lake Erie – sensitivity to convection, microphysics and the water temperature. Advances in Science and Research, 4(1), 15–22. https://doi.org/10.5194/asr-4-15-2010
Topics in Lake Effect Snow Forecasting. (n.d.). Retrieved September 24, 2022, from https://www.meted.ucar.edu/norlat/snow/lake_effect/navmenu.php
Tymvios, F., Charalambous, D., Michaelides, S., & Lelieveld, J. (2018). Intercomparison of boundary layer parameterizations for summer conditions in the eastern Mediterranean island of Cyprus using the WRF - ARW model. Atmospheric Research, 208, 45–59. https://doi.org/10.1016/j.atmosres.2017.09.011
Vogelezang, D. H. P., & Holtslag, A. A. M. (1996). Evaluation and model impacts of alternative boundary-layer height formulations. Boundary-Layer Meteorology, 81(3–4), 245–269. https://doi.org/10.1007/BF02430331
Welle (www.dw.com), D. (n.d.). Greece: Rare snowstorm leaves 3 dead | DW | 16.02.2021. DW.COM. Retrieved July 24, 2022, from https://www.dw.com/en/greece-rare-snowstorm-leaves-3-dead/a-56592957
Wilks, D. S. (2019). Statistical methods in the atmospheric sciences (4th ed). Elsevier.
WRF Users’ Guide. (2020). https://www2.mmm.ucar.edu/wrf/users/docs/user_guide_v4/v4.2/contents.html
WWRP/WGNE Joint Working Group on Forecast Verification Research. (2015, January 26). https://www.cawcr.gov.au/projects/verification/#Contributors_to_this_site
Yavuz, V., Deniz, A., Özdemir, E. T., Kolay, O., & Karan, H. (2021). Classification and analysis of sea‐effect snowbands for Danube Sea area in Black Sea. International Journal of Climatology, 41(5), 3139–3152. https://doi.org/10.1002/joc.7010
Zhang, M., & Song, H. (2006). Evidence of deceleration of atmospheric vertical overturning circulation over the tropical Pacific. Geophysical Research Letters, 33(12). https://doi.org/10.1029/2006GL025942
Αθανασίου, Φ. Α. (2019). Βελτιστοποίηση της αναπαράστασης των διεργασιών του οριακού στρώματος σε αριθμητικό μοντέλο πρόγνωσης καιρού. https://doi.org/10.26262/HEAL.AUTH.IR.307206
Ευσταθίου, Γ. (2013). Μελέτη των παραμετροποιήσεων του ατμοσφαιρικού οριακού στρώματος και της μικροφυσικής στην προσομοίωση ισχυρών βροχοπτώσεων [Πανεπιστήμιο Ιωαννίνων, Σχολή Θετικών Επιστημών, Τμήμα Φυσικής, Τομέας Αστρογεωφυσικής, Εργαστήριο Μετεωρολογίας και Κλιματολογίας]. https://doi.org/10.12681/eadd/36971
Ζιακόπουλος, Δ. (2009). Καιρός, ο γιός της Γης και του Ήλιου.: Vol. Τόμος 2ος. Ιδιωτική Έκδοση.
Ζιακόπουλος, Δ., & Φραγκούλη, Π. (2015). 7.1 Θερμικός Άνεμος. In ΤΟ ΕΓΧΕΙΡΙΔΙΟ ΤΟΥ ΜΕΤΕΩΡΟΛΟΓΟΥ - ΠΡΟΓΝΩΣΤΗ (pp. 117–126). ΕΘΝΙΚΗ ΜΕΤΕΩΡΟΛΟΓΙΚΗ ΥΠΗΡΕΣΙΑ.
Καραγιαννίδης, Α., & Λαγουβάρδος, Κ. (2021). Κακοκαιρία «Μήδεια»: Στους -20 βαθμούς Κελσίου η ελάχιστη θερμοκρασία – Ριπή ανέμου κοντά στα 120 χλμ/ώρα. meteo.gr - Προγνώσεις καιρού για όλη την Ελλάδα. https://www.meteo.gr/www.meteo.gr?entryID=1639
Καρακώστας, Θ. (2020). Σημειώσεις Δυναμικής Μετεωρολογίας. Πρόγραμμα Μεταπτυχιακών Σπουδών στην “Μετεωρολογία, Κλιματολογία και Ατμοσφαιρικό Περιβάλλον.” Αριστοτέλειο Πανεπιστήμιο Θεσσαλονίκης, Σχολή Θετικών Επιστημών, Τμήμα Γεωλογίας
Κολυβά - Μαχαίρα, Φ., & Μπόρα - Σέντα, Ε. (2014). Στατιστική. Θεωρία και Εφαρμογές. Εκδόσεις Ζήτη.
Παπαπέτρου, Ά., & Καραταράκης, Ν. (2021). Χιονοπτώσεις Στην Αττική 15-17 Φεβρουαρίου 2021- Κλιματικά Στοιχεία. Εθνική Μετεωρολογική Υπηρεσία (ΕΜΥ), 5.
Πυθαρούλης, Ι. (2020a). Σημειώσεις Αριθμητικής Πρόγνωσης Καιρού. Πρόγραμμα Μεταπτυχιακών Σπουδών στην “Μετεωρολογία, Κλιματολογία και Ατμοσφαιρικό Περιβάλλον.” Αριστοτέλειο Πανεπιστήμιο Θεσσαλονίκης, Σχολή Θετικών Επιστημών, Τμήμα Γεωλογίας
Πυθαρούλης, Ι. (2020b). Σημειώσεις Συνοπτικής Μετεωρολογίας. Πρόγραμμα Μεταπτυχιακών Σπουδών στην “Μετεωρολογία, Κλιματολογία και Ατμοσφαιρικό Περιβάλλον.” Αριστοτέλειο Πανεπιστήμιο Θεσσαλονίκης, Σχολή Θετικών Επιστημών, Τμήμα Γεωλογίας
Ρούση, Ε. Μ. (2008). Συμβολή στη μελέτη της θερμοκρασίας της Μεσογείου με τη χρήση στοιχείων περιοχικών μοντέλων κυκλοφορίας. Μεταπτυχιακή Διατριβή Αριστοτέλειο Πανεπιστήμιο Θεσσαλονίκης, Σχολή Θετικών Επιστημών, Τμήμα Γεωλογίας https://doi.org/10.26262/HEAL.AUTH.IR.108094
Χατζούδης, Χ. Γ. (2018). Μελέτη της ευαισθησίας ενός αριθμητικού μοντέλου πρόγνωσης καιρού στην παραμετροποίηση της ανοδικής μεταφοράς. Μεταπτυχιακή Διατριβή Αριστοτέλειο Πανεπιστήμιο Θεσσαλονίκης, Σχολή Θετικών Επιστημών, Τμήμα Γεωλογίας https://doi.org/10.26262/HEAL.AUTH.IR.302237
Εισερχόμενη Αναφορά
- Δεν υπάρχουν προς το παρόν εισερχόμενες αναφορές.